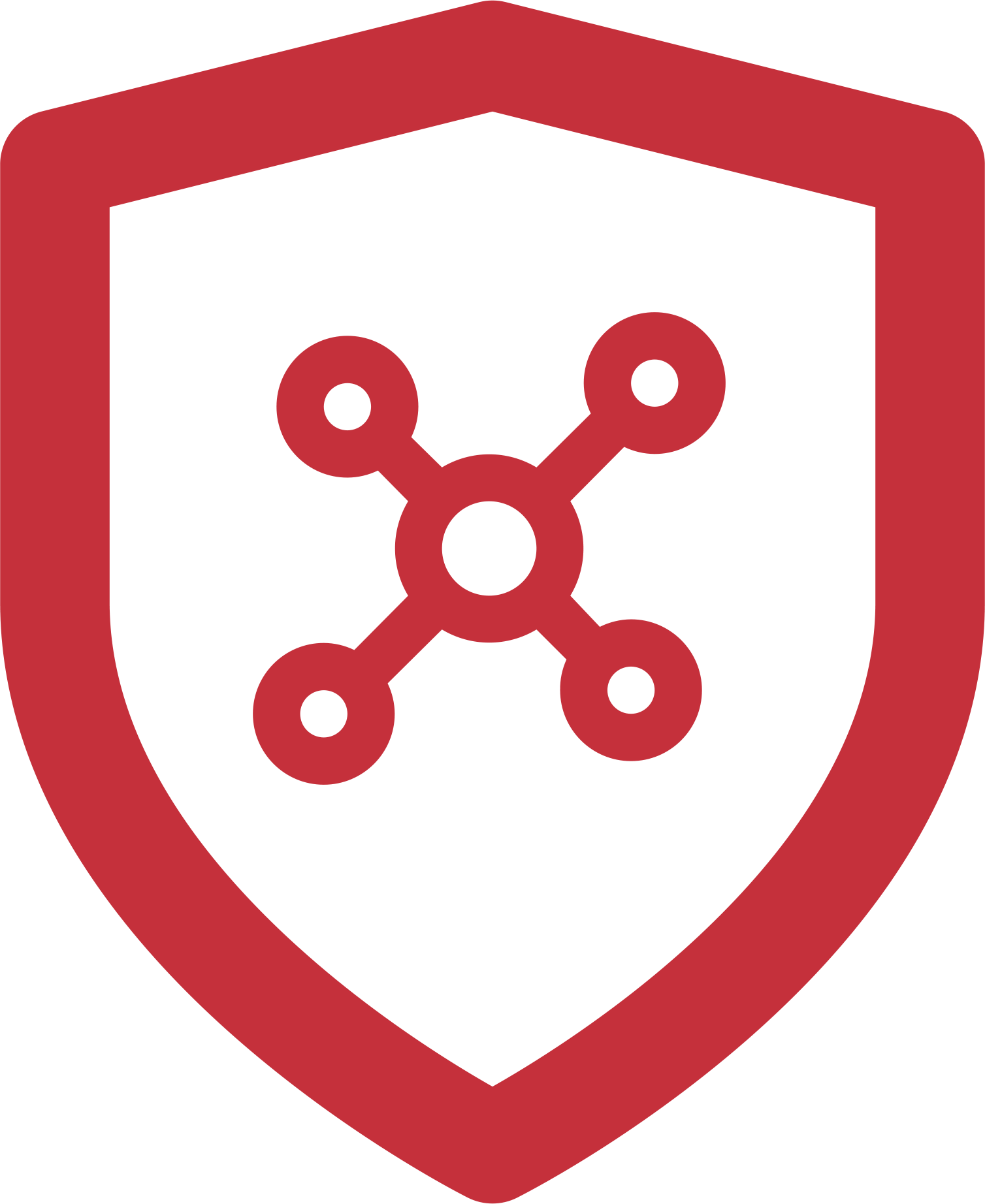
Lifeboat Foundation NanoShield Version 0.90.3
IN MEMORY OF CASSIE FREITAS
By Michael Vassar, Head of our Long-Term Strategy Division, and Robert A. Freitas Jr., Head of our Nanomedicine Division, with participation by Amara D. Angelica, Philippe Van Nedervelde, Mike Treder, and other Scientific Advisory Board members. This is an ongoing program so you may submit suggestions to [email protected].
Overview
The most immediate danger facing life on earth is probably that posed by biological weapons and emergent disease. The Lifeboat Foundation BioShield proposal [1], described by Lemelson-MIT Prize winner Ray Kurzweil and U.S. Senate majority leader Bill Frist is our recommended response to this danger. The BioShield proposal emphasizes the development of technologies to combat bioweapons, such as biological viruses, by developing broad tools to prevent their development and to destroy them.
However, tomorrow’s biggest danger is nanoweapons and we believe it is now time to develop a solution to this problem. Kurzweil [13] said
“As the threshold for self-organizing nanotechnology approaches, we will then need to invest specifically in the development of defensive technologies in that area, including the creation of a technological immune system.”
There are two types of nanoweapons:
- Self-replicating weapons (e.g., ecophages) that make copies of themselves; their only means of attack may be to “eat” the enemy or his resources as they self-replicate.
- Nonreplicating nanoweapons, similar to the tools of war today, that are manufactured in a factory and then used in battle.
The NanoShield proposal has been designed primarily to handle self-replicating weapons, but it will also be an excellent first line of defense against nonreplicating weapons. Nonreplicating weapons are more difficult to defend against, since they don’t need to spend a lot of time and effort in replicating. They are also easier to design, since they do not have to include instructions on how to replicate.
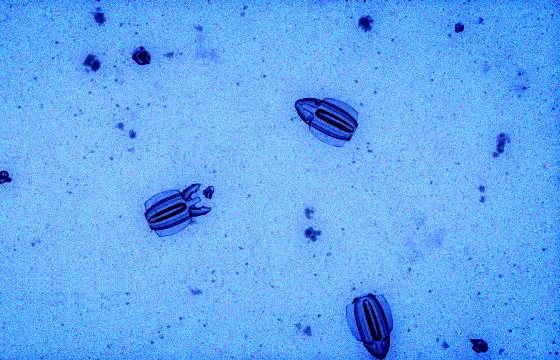
Artist’s rendering of self-replicating nano robots. Courtesy, E-spaces
Background
One of the earliest-recognized and best-known dangers of molecular nanotechnology is the risk that artificial nanotech replicators [2] — capable of digesting biological materials and functioning autonomously in the natural environment — could quickly convert the entire global ecosystem into more copies of themselves. This is a scenario often referred to as “grey goo”, but more accurately termed “global ecophagy”, a term coined by Robert A. Freitas, Jr. [3].
Such replicators, called “ecophages”, would constitute a class of sophisticated artificial life forms more lethal than any plague that has ever existed on this planet. If they are ever built and released, ecophages will need to be controlled by a sophisticated artificial immune system more powerful than any immune system that has appeared in natural biology. The human body has not evolved any natural immunity against mechanical replicators. We must invent this immunity ourselves.
The human immune system does not have to recognize dangerous invaders the way a nanotech system would. Our immune system merely has to recognize non-invaders and attack everything else. Another important distinction is that biological immune cells — and the invaders that they must combat — will both replicate at biological speeds and energy levels.
In contrast, a contest of exponentially growing numbers of nanotech devices would cook the biosphere in waste heat [3], especially if a large number of novel replicating nanodevices were released simultaneously, and if a different type of defensive device were needed to stop each of them.
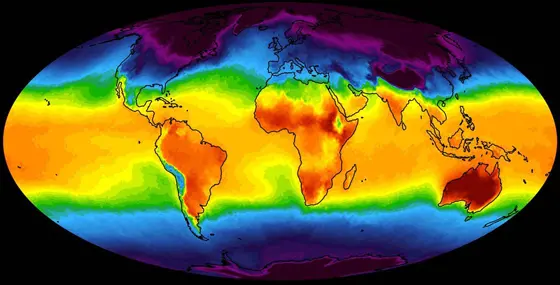
Average Earth surface temperature for January 2003. Courtesy, NASA.
The human immune system also benefits from co-evolution with its assailants. Microbes are selected to not overwhelm it too quickly, otherwise the victim would die and the microbes would also lose. The greatest immunological advantage of a human immune system may be the vast number of humans in which it is found. When microbes overwhelm it in one human that human’s selfish genetic material can afford to simply die, while living on in other humans. But we have only one earth, so we cannot afford such sacrifices of a global scale.
For these reasons, we have a more difficult task than that facing nature if we are to defend ourselves against artificial replicators. But we also have advantages not possessed by nature. Most important among these are the power to use design, to thoroughly analyze nanomachines we find, and to employ macroscale phenomena in our defense.
The NanoShield Proposal
Our proposal for a NanoShield encompasses five specific recommendations, as follows.
1. Threat Detection
To begin thinking about this problem, it is first necessary to determine the incidence of ecophagy that is likely to be detectible. This will be primarily a function of the pervasiveness of our defenses, and of the efficiency with which they can identify ecophagy, bearing in mind that ecophages may be intentionally designed to resist identification.
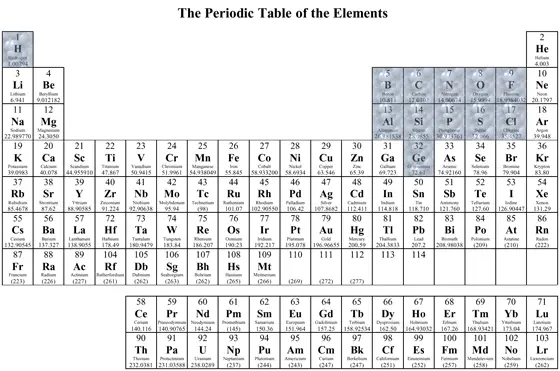
Pure crystals of diamond are brittle and easily fractured. The intricate molecular structure of a diamondoid nanofactory product will more closely resemble a complex composite material, not a brittle solid crystal.
Most diamondoid materials used for nanomachinery would be constructed from the atoms of 12 elements in the Periodic Table: carbon (C), silicon (Si) or germanium (Ge) in Group IV, nitrogen (N) or phosphorus (P) in Group V, oxygen (O) or sulfur (S) in Group VI, fluorine (F) or chlorine (Cl) in Group VII, boron (B) or aluminum (Al) in Group III, and, of course, hydrogen (H).
Courtesy, Nanofactory Collaboration.
If nanorobots were withdrawing primarily carbon from the environment to build diamond, you could in principle search for the surplus or “waste” atoms that they discharge. For example, if an ecophage was consuming CHON-based organic material, and removing mainly the C atoms for incorporation into its mostly hydrocarbon-based replicas, it would presumably be discharging the unused H, O, and N atoms into the local environment as waste products in some form.
But trying to detect ecophages by searching for the waste atoms offers several challenges:
If they discharge as they feed, the discharge could be hidden by designing the ecophage to release mostly “natural” appearing effluents — for example, with waste O, N and H atoms being released as atmosphere-like O2, N2, H2, or H2O.
Unless there were a lot of ecophages concentrated in a small area, the volume of such effluent discharges would be relatively small, and any wind could rapidly disperse the effluents, even if they could somehow be recognized as artificial.
Ecophages could package their wastes into compressed-gas or solid-matter pellets and then drop them into the dirt. If they were covered with a camouflage coating, these droppings would be undetectable.
Some ecophages might be made of nonhydrocarbon ceramics such as boron nitride or silicon nitride, and thus would have a different effluent signature than expected for diamond ecophages. Such devices might not even need to consume biology during their relatively slow Build (replicating) phase, but could consume rocks etc. instead, and then only consume biology during their relatively fast Destroy (nonreplicating) phase [3].
If the ecophage made a good effort to camouflage its effluents they probably could not be detected, so a different detection method would be needed to find the ecophage.
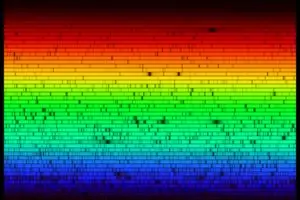
Extremely high resolution spectrum of the Sun showing thousands of elemental absorption lines.
Two possible techniques are spectrographic analysis and sonographic detection. One could detect MM products spectroscopically based on the presence of particular types of chemical bonds. However, this could be defeated by ecophage designers by coating them with something that looks natural, like silica (sand) or an outer shell of some magnesium-iron-silicate-etc. mineral that looks exactly like ordinary dirt.
MM products could be detected sonographically based on their existence in the environment as multiple clusters of matter that resonate at the same set of frequencies. However, this would have the problem that nanorobots and their parts have very high resonant frequencies — gigaHertz or teraHertz — because they are so small. Acoustic waves of these frequencies are hugely attenuated in their passage through air, or even water, so their useful range would be very short, on the order of microns. And it would not be wise to assume that ecophages will cluster into nice large macroscale “tuning forks” that would make them easier to detect — an ecophage designer would probably not require his ecophages to do any aggregation at all in order to replicate.
Another method is to examine chunks of material. If we assume a possible size on the order of 10 cubic microns, detection of a potentially dangerous nanodevice in a cubic meter of material would require that 1017 chunks of material be examined. To perform such an examination for each of the 1015 cubic meters within two meters of the earth’s surface using “rod logic” (nanomechanical computation at the molecular level, as proposed by K. Eric Drexler in Nanosystems) would require roughly the Earth’s incident solar energy over a 15 minute period for every computational operation involved in characterizing a particle as a threat.
It would also require the practical disassembly of every object on earth and some technique for utilizing the information acquired. Because some defensive probes would be expected to fail in the ordinary course of events, this technique would also fail to detect ecophagy armed to defend themselves. Even a less thorough examination, testing a single 10 cubic micron sample volume chosen randomly in each 10 cm3 region of space (a one-in-a-trillion sampling rate), would be disruptive, computationally intensive, and relatively easy to circumvent.
Another method is to use three-dimensional images of nanoparticles that have been obtained with a microscope using newly discovered coherent X-ray diffraction instead of focusing them [12]. This would allow for non-destructive forensic analysis to see if it is an MM product. Shielding could frustrate this process.
Trying to detect MM products tactilely, based on hardness, could be frustrated by camouflage coatings and would require physical contact, which generates a large-numbers problem.
So rather than computationally analyzing and characterizing random chunks of matter to determine whether they are capable of self-replication, a better solution is to continuously monitor the heat signature of the entire global surface and possibly the subsurface [3]. If this is combined with sophisticated pattern recognition, developing trouble will be detected reasonably quickly. Manual inspection nanorobots would then be sent to only those regions identified by the thermal pattern recognition software as scoring high on the “possible trouble” index. The atmosphere and oceans will need to be monitored as well.
Is it possible for an ecophage to mask its infrared signature and thus elude detection? Mechanical and chemical activities develop waste heat, so eventually this heat must appear somewhere in the environment. One strategy an ecophage could employ to evade detection would be to transfer the heat from its site of activity to some distant site, where the heat could be dispersed more widely and thus would be harder to distinguish from background levels.
For example, a Peltier-effect cooling system (electronic refrigeration) could transfer heat from the ecophages through an underground wiring network to a distant distributed thermal radiator system, possibly diluting the thermal signature by 1000:1 or more. Fluid-driven heat pipes or a complex of fractal diamond pipes (diamond is an excellent heat conductor) might also be effective. Another ecophagic strategy might be to acquire the biological feedstock at a given surface site but not process it there, transporting it instead to a distant location where the thermal signature of chemical processing could be better disguised — for example, in a processing facility located deep underground.
All such approaches can be defeated if a high-resolution, high-sensitivity global thermal map has been created, good baseline statistics have been collected for many years for both surface and subsurface temperatures, and they are closely and continuously monitored using sophisticated pattern-recognition software.
There is one approach that might defeat infrared monitoring: stealth. Summarizing a key point from Freitas’ ecophagy paper [3], Kurzweil [13] notes:
“We can envision a more insidious possibility. In a two-phased attack, the nanobots take several weeks to spread throughout the biomass but use up an insignificant portion of the carbon atoms, say one out of every quadrillion (1015). At this extremely low level of concentration, the nanobots would be as stealthy as possible [Freitas’ “Build” phase]. Then, at an “optimal” point, the second phase would begin with the seed nanobots expanding rapidly in place to destroy the biomass [Freitas’ “Destroy” phase]. For each seed nanobot to multiply itself a quadrillionfold would require only about 50 binary replications, or about 90 minutes.”
Therefore for additional safety, some random sampling of materials should be done, in addition to simply monitoring heat signatures. Only a thorough examination (including partial disassembly) of found objects will suffice to determine whether they are products of molecular manufacturing (MM) or not — although a variety of tests short of disassembling the object in question could prove useful as well.
2. Non-Specific Immunity Defenses
Instrumentalities should be put in place that constitute a general, nonspecific response to any perceived ecophagic threat. For example, inspection nanorobots could be deployed to any area that is suspected of having any sign of possible ecophagic activity.
If ecophages are detected, there could be a response from prepositioned stores of generic defensive nanorobots manufactured by a global network of defensive nanofactory stations that have been put in place well in advance of the outbreak of the threat. These first-line defensive nanorobots will have generic abilities to disable ecophages — e.g., sensor blinding, spray painting to ruin energy-producing solar cells, and perhaps some capability of mechanical disassembly or physical crushing, electric shock, e-beam irradiation, and so forth. These defenses will buy time for the specific immunity defenses to kick in.
3. Specific Immunity Defenses
A second set of instrumentalities that should be put in place comprise a specific, targeted immunity response to a perceived ecophagic threat. These defenses would be designed to attack the particular ecophage in question. They could not be launched until the ecophage was identified and its weaknesses determined. A regular program of collecting and inspecting nanorobots found in the environment via sampling from randomly selected locations would help to establish a statistical baseline on extant nanorobot populations and would also provide an early warning of new nanorobotic capabilities that are being fielded.
The ability to detect and identify an object implies the ability, if necessary, to selectively deliver energy into that object. Ultrasound in the proper resonant frequency could deliver destructive amounts of energy into pre-specified and molecularly precise objects. Specific surface chemistries can be attacked via the relevant chemical reactions. Chemical bonds can also be broken by electromagnetic quanta at the correct frequency.
For example, an ecophage that internally employed mechanosynthetic tooltips possessing Ge-C bonds might be disabled by exposure to 21 THz infrared radiation, the approximate resonant stretching frequency of the Ge-C dimer bond. Similarly, exposure to GHz microwaves might pump unplanned energy into purely mechanical components of nanorobots such as the logic rods in mechanical nanocomputers operating at GHz frequencies, thus permanently damaging them, if these moving components incorporate any unbalanced electrical charge or conductive pathways in their molecular structure.
A methodology similar to that used by the human immune system is another option. Surfaces complementary to those of undesirable environmental contaminants, including MM devices, can be created and used to selectively bind MM devices and isolate them. Sensors, solar cells and other key parts of an MM device can be targeted as well. Such specific anti-MM defenses would be launched at a detected infection, since it is, of course, unlikely that permission would be given to carpet the entire Earth (and its atmosphere and oceans) with them.
While molecular manufacturing systems must fight entropy to build molecularly precise systems, countermeasures can work with entropy. In other words, on the molecular level, as on any other, once detection has occurred, destruction is far easier than creation and takes much less time [3]. As a result of this, except for ecophagic populations much greater than the populations of countermeasure devices, the time taken by countermeasures to eliminate ecophagic infestations will be dominated by search time.
Search time should usually be inversely proportional to the concentration of targets. For this reason, an exponentially replicating ecophage population can be stopped by a constant-sized population of anti-ecophages, or more precisely, a constant concentration (per volume) of anti-ecophages. This implies two things: (a) You don’t need to respond to an ecophage outbreak instantly; and (b) You don’t need to get into an exponential race.
As MM populations in the environment are monitored, any non-Brownian diffusion or rapid increase in incidence should flag the attention of authorities who might examine the relevant population data, schemata of the threatening nanodevice, and simulations of the device’s behavior. If they are concerned, they should authorize the release of countermeasures stockpiled by a large and diffuse planetary grid of nanofactories. Countermeasures need not be self-replicating, and in fact should not be, since that would require them to be complex and slow and in addition, would raise the possibility that they could be preempted for use as ecophages.
Division of labor is generally efficient, and the production of countermeasures by specialized productive systems is an example of this. Although a grid of productive nanofactories must be created and stocked with feedstock and energy ahead of time for countermeasure production, specific countermeasures need not be created until replicators pose an immediate danger, so long as the total productive capacity available for countermeasure production is sufficiently great.
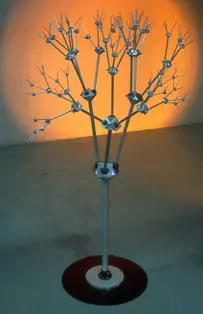
Manually constructed passive articulated bush robot model.
Countermeasures could take the form of small molecules, nanomachines, or macroscale devices such as ultrasound generators, sorting devices, or even bush robots with target specific branch tips. (“Bush robots” will have an immovable base that repeatedly branches in a fractal way into trillions of nanoscale fingers. [4]) Collectively, specific countermeasures can be seen as the equivalent of specific immunity. It could be innocuous, automatic, and continuous. But unlike specific immunity in biology, ecophage countermeasures can be subject to higher level analysis and centralized control, enabling their modification to correct any unintended damage.
4. Emergency Defenses
A third set of instrumentalities should be put in place that constitute broad-brush emergency responses to a larger ecophagic threat. We cannot rule out the possibility of rare situations in which the normal nonspecific defenses fail, and successful specific defenses cannot be mobilized. Examples of such a dire emergency would be the existence of ecophagic replicators too numerous for cleanup or the recognition that an uncharacterized ecophage or one with no known specific countermeasures is replicating unexpectedly rapidly.
In such cases, it would be helpful if the NanoShield included emergency defenses that would be effective against a wide range of ecophagy types. With even broader impact than non-specific immunity responses, the use of emergency defenses would disrupt lives and economic and ecological systems. But the mere existence of these relevant defenses, prepared but unused, will not cause harm.
Many of the proposed emergency responses will themselves cause additional damage during the process of halting the ecophagic outbreak, much as a surgeon’s scalpel damages the tissue through which it cuts during an operation to remove a more life-threatening tumor. For this reason, emergency responses should be considered a last resort and should be activated only in the direst of circumstances.
In the aftermath, advanced molecular manufacturing and nanomedicine should allow us to repair many forms of damage to biological organisms, including individual human beings. Much, although perhaps not all, of the natural global ecological infrastructure might be reconstituted if proper genetic and statistical records have been maintained that describe the location and design of every large object and organism.
The possible misuse of specific countermeasures or emergency defenses is inevitably a serious concern, but one that should be almost as manageable as the risk of misuse of nuclear weapons. We say only “almost as manageable” because MM seems to favor evasion more than detection. This makes infiltration of the defensive systems themselves easier than it is in the case of nuclear weapons.
Also, unlike nuclear defense monitoring systems, such as Geiger counters, anti-ecophagy defense systems leave footprints when taking in information from the outside world, in the form of the data stream from their continual monitoring activities.
Some examples of emergency ecophagy defenses are:
- Skysweepers. Air-filtering nanoscoop devices could filter the whole Earth’s atmosphere, thereby removing all aerovores, as first described by Freitas [3].
-
Artist’s rendition of a 100 micrometer foglet. Utility Fog. Massive utility fog curtains capable of establishing filters for the separation of the atmosphere into compartments, containing an ecophagy outbreak or enabling the rapid establishment of expanding sterile bubbles (barriers within which organisms can be safe from any ecophagy that they don’t bring in with them).
Curtains may defend their integrity with multiple layers, with sensors that can recognize damage and respond with films of relatively inert substances that cannot be modified by known mechanochemical reactions (at room temperature or in general).
The originator of the Utility Fog concept was J. Storrs “Josh” Hall. Read his original writeup Utility Fog: The Stuff that Dreams are Made of.
-
Artist conception of giant sun shades orbiting Earth. Courtesy, BBC. Solar Shades. Large solar shields that can be used to block the sunlight reaching Earth’s surface, thus denying power or reducing the power available to solar-powered replicators. Prompt disablement or sequestration over a short time period of ecophages rendered dormant or lethargic may allow most terrestrial plant life to survive the prolonged darkness unharmed.
-
Localized Heating. Localized heating increases thermal motions in the mechanosynthetic tools used by the ecophage to build new molecular structures, causing onboard temperature-sensitive mechanosynthetic reactions to become unreliable. This would lead to fatal errors in the fabrication and assembly of daughter ecophages and most probably the permanent poisoning of the onboard mechanosynthetic tools. Localized heating may be an inevitable side effect of the use of other specific countermeasures at high power but can also be obtained more directly by relatively simple measures.
For example, an orbiting mirror could be used to focus concentrated sunlight into a specific ecophagic outbreak region, with the duration and intensity of localized temperature increases carefully controlled to maximize damage to the ecophages and minimize damage to the environment. Alternatively, an orbiting laser beam could be directed onto the ecophagic outbreak site (heating the ecophages). Ideally any temperature changes would be confined to the smallest possible area.
-
Electromagnetic Pulse (EMP). Nuclear weapon explosions are known to create very sharp pulses of high-intensity electromagnetic radiation that can destroy electronic equipment. EMP can also be generated by non-nuclear systems. Ecophages with onboard nanoelectronic components, including sensors, computers, electrical motors or generators, and power conduits, would be seriously damaged and probably rendered entirely inoperative, if exposed to EMP. Only ecophages with all-mechanical inner workings or that are heavily shielded would be immune to EMP damage.
Of course, many microelectronic and macroelectonic devices that are not “hardened” (shielded and otherwise protected against radiation) will be similarly damaged and would have to be rebuilt in the aftermath, although EMP generators could be deployed against ecophagic outbreaks in limited areas, using directional antennas to minimize damage to electronic devices. One important benefit of this approach is that EMP could be used against ecophages infesting populated areas, without causing significant biological damage to living things.
-
Radiation hazard symbol. Radiation. Finally, high-power emitters of fairly penetrating radiation, possibly x-rays or electrons from thermionic emitters, can be used to destroy all large molecularly structured systems within a large volume. Radiation can be tuned to minimize interaction with organic tissue, particularly with key tissues such as the nervous system, but basically this proposal relies on nanomedical systems that can be rapidly deployed to repair nanoscale damage before it brings about larger scale and more complex forms of damage.
This proposal may work well with (b), enabling organisms to be sterilized while they enter quarantined compartments. Other methods of sterilization include the use of nanomachines to remove all molecules from an organism’s body that are not pre-characterized as “normal”. This proposal is fairly similar to a generalized version of what human immune systems typically try to accomplish, e.g., the removal of everything except an enumerated list of molecule types, so the immune system might actually be enlisted to aid in the identification of nanosystems that natural immune cells have no way to attack. Biocompatible surfaces are likely to be well characterized in nanomedicine, so such surfaces can probably be identified by cleanup nanomachines unless the ecophages have masked surfaces to evade detection.
5. New Monitoring Agencies
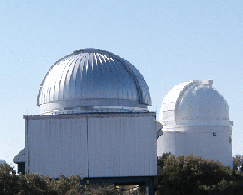
The Spacewatch 1.8-meter and 0.9-meter telescopes on Kitt Peak, 45 miles southwest of Tucson, Arizona.
Each government participating in the NanoShield should establish and fund a new monitoring agency analogous to existing governmental agencies that already monitor outbreaks of computer viruses — most notably the U.S. Department of Homeland Security’s Computer Emergency Readiness Team (US-CERT), the world’s premier public-sector computer security monitoring agency [5]. Other analogous monitoring efforts include the Tsunami Warning System [6] operated by NOAA and the U.S. National Weather Service, and the Spaceguard [7] telescopic monitoring effort, which continuously searches the skies for evidence of an approaching asteroid capable of impacting the Earth.
The proposed new nanotech monitoring agencies would be charged with initiating the early studies and preliminary implementation of the NanoShield. Each country’s agency should coordinate with the other agencies and when they are ready to establish active defenses outside their own countries, they should establish a lead body to handle this.
The ultimate objectives of these nanotech monitoring agencies, as originally noted by Freitas [3], would be
Initiating a long-term research program designed to acquire the knowledge and capability needed to counteract ecophagic replicators, including scenario-building and threat analysis with numerical simulations, measure/countermeasure analysis, theory and design of global monitoring systems capable of fast detection and response, IFF (Identification Friend or Foe) discrimination protocols, and eventually the design of relevant nanorobotic systemic defensive capabilities and infrastructure.
A related long-term recommendation is to initiate a global system of Comprehensive in situ ecosphere surveillance, potentially including possible nanorobot activity signatures (e.g. changes in greenhouse gas concentrations), multispectral surface imaging to detect disguised signatures, and direct local nanorobot census sampling on land, sea, and air, as warranted by the pace of development of new MM capabilities.
This would lead to various practical early-stage monitoring activities that could be implemented today, including most importantly [3]:
Continuous comprehensive infrared surveillance of Earth’s surface by geostationary satellites, both to monitor the current biomass inventory and to detect (and then investigate) any rapidly-developing artificial hotspots. This could be an extension of current or proposed Earth-monitoring systems (e.g., NASA’s Earth Observing System [8] and disease remote-sensing programs [9]) originally intended to understand and predict global warming, changes in land use, and so forth — initially using non-nanoscale technologies. Other methods of detection are feasible and further research is required to identify and properly evaluate the full range of alternatives.
Unstable Arms Race: Nonreplicating Nanoweapons
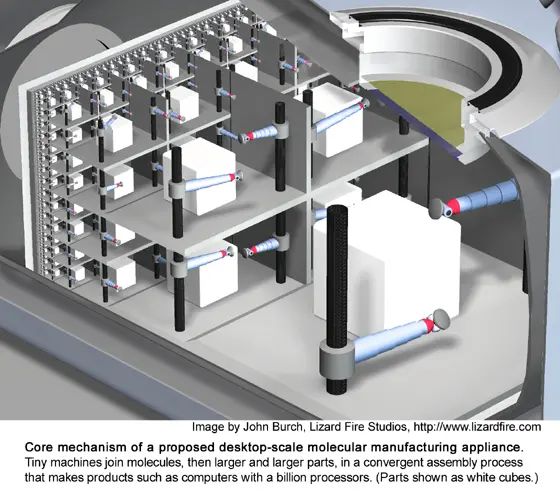
Molecular manufacturing also raises the possibility of horrifically effective nonreplicating nanoweapons. The difference in purpose between a nanotech weapon and an ecophage is that an ecophage seeks primarily to replicate by consuming biological matter, thus becoming a direct resource competitor to biology, while nanotech weapons can have a far greater diversity of purposes, including killing only specific parties.
Ecophages must devote significant resources to replication, whereas nanoweapons can focus solely on destruction. This means that active nanoweapons can be far more dangerous per gram than ecophages, and can act much more rapidly because they need not waste time replicating.
As an example, the smallest insect is about 200 microns. This creates a plausible size estimate for a nanotech-built antipersonnel weapon capable of seeking and injecting toxin into unprotected humans. The human lethal dose of botulism toxin is about 100 nanograms, or about 1/100 the volume of the weapon. As many as 50 billion toxin-carrying devices — theoretically enough to kill every human on earth — could be packed into a single suitcase.
Guns of all sizes would be far more powerful, and their bullets could be self-guided. Aerospace hardware would be far lighter and higher performance. Built with minimal or no metal, it would be much harder to spot on radar. Embedded computers would allow remote activation of any weapon, and more compact power handling would allow greatly improved robotics.
Other possible nanoweapons (most of which have known defenses that could be incorporated into NanoShield) include:
Arbitrarily large numbers of any robot.
Deuterium filters for separating deuterium from seawater.
Microscale isotopic separation of uranium.
Massive utility fog banks that simply contain all movement in a large region.
Computer viruses that make other people’s nanofactories build bombs.
Inhalable or skin-penetrating machines that travel to the nervous system, allowing outside sources to take over inputs or outputs.
Massive nanofactories could consume a substantial fraction of earth’s CO2.
An important question is whether nanotech weapons — both replicating and nonreplicating — would be stabilizing or destabilizing. Nuclear weapons, for example, could perhaps be credited with preventing major wars since their invention. However, nanotech weapons differ from nuclear weapons. Nuclear stability stems from at least three factors. The most obvious is the massive destructiveness of all-out nuclear war.
All-out nanotech war is probably equivalent in the short term, but nuclear weapons also have a high long-term cost of use (fallout, contamination) that would be much lower with nanotech weapons. Nuclear weapons cause indiscriminate destruction; nanotech weapons could be targeted. And nuclear weapons require massive research effort and industrial development, which can be tracked far more easily than nanotech weapons development.
Finally, nanotech weapons can be developed much more rapidly due to faster, cheaper prototyping. Greater uncertainty of the capabilities of the adversary, less response time to an attack, and better targeted destruction of an enemy’s visible resources during an attack all make nanotech arms races less stable. Also, unless nanotech is tightly controlled, the number of nanotech nations in the world could be much higher than the number of nuclear nations, increasing the chance of a regional conflict blowing up.
Bottom line: all problems that could be caused by nanotech weapons might not be solvable by the NanoShield alone, but having the NanoShield in place would provide an excellent first line of defense. We welcome suggestions from the public on how to improve the NanoShield so it can better handle nonreplicating nanoweapons.
Risks of NanoShield
The risk that the NanoShield would malfunction and accidentally destroy property or life on this planet can be made as close to zero as desired by increasing the reliability and redundancy of control systems. The greater and true risk of NanoShield implementation is that it might be purposely abused by people. For example, a NanoShield in malevolent hands could be used to oppress individuals, groups, or entire countries.
To minimize this risk, authority to activate the NanoShield should be distributed among as many responsible but competing interests as is practical, consistent with the need for potentially rapid decision making by parties who have demonstrated by past practice that they are ready and willing to take decisive action if the need arises.
One good solution might be to have the NanoShield controlled by a coalition of democracies, perhaps NATO. Less ideal would be to vest control of the NanoShield in the hands of a single strong democracy such as the United States or Australia. A more dangerous outcome may occur if all democracies ignore this vital issue and allow, by default, a dictatorship such as China, or a small private group, or even a lone individual, to control the NanoShield.
It is unlikely that the UN can effectively administer the NanoShield due to structural problems including its inability to make rapid decisions, the veto power of non-democratic nations having permanent seats on the Security Council, and the large number of dictatorships represented among the UN membership.
SecurityPreserver Proposal
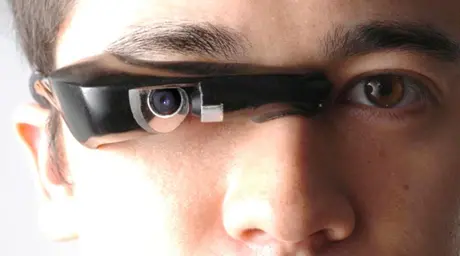
Sousveillance as a situationist critique of surveillance. This wearable device allows you to “watch the watchers”.
To further increase global security and to lessen the need for the most dangerous elements of the NanoShield to be activated, we recommend consideration of the Lifeboat Foundation SecurityPreserver proposal [10], to be implemented alongside the NanoShield.
The SecurityPreserver would deal with the problem that a powerful nanoweapon could be developed in secret that could wipe out life on the earth before the NanoShield could deal with it.
The SecurityPreserver system would consist of multiple parallel, globally deployed, nanotechnology-based surveillance systems, such as “smart dust” (micro- or nanosize networked sensors that could covertly detect anything). In addition, “sousveillance” systems would be used. These would enable the public to turn the tables and monitor the government (and perhaps others) via tools like smart-dust data feeds — a possible checks-and-balance system for the coming nano age.
Transparency vs. Privacy
Of course, a smart dust system could also be used to abridge long-held Constitutionally-protected rights to privacy. Special enabling legislation or even an Amendment to the U.S. Constitution might be required to implement smart dust in a manner that would pass Constitutional muster at the U.S. Supreme Court with similar need for changes in laws of other countries. But as noted by Neil Jacobstein, Chairman of the Institute for Molecular Manufacturing: “Nanotechnology-enabled transparency and accountability will produce the worst form of government, except for all those other forms that have been tried from time to time.”
Is it acceptable for governments to monitor civilians with quintillions of sensors, and for civilians to monitor their governments with quintillions of sensors? Or is that irresponsible and dangerous? A larger policy question is: should the NanoShield try to handle every possible class of nanoattack, or should we attempt to stop hostile forces from unleashing the attacks in the first place — which may require extensive surveillance? While NanoShield appears sufficiently robust to confront most ecophagy attacks, there is no guarantee that it can handle all conceivable types of nanoweapons. Each attack prevented by good surveillance is one less attack that might possibly overwhelm the NanoShield. Thus the SecurityPreserver, if implemented, may significantly reduce the total number of threats that the NanoShield may be asked to confront.
Even combining smart dust with the three-layer defensive system proposed for NanoShield cannot provide an absolute guarantee of safety against all possible nanotech threats, especially given the power that personal nanofactories [11], which could be acquired by individuals, including terrorists. But NanoShield should provide an excellent first line of defense, and adding smart dust would further strengthen it.
Conclusion
Any particular ecophagy or nonreplicating nanoweapon defense can be circumvented, but the number of people proposing ecophagy defenses is likely to exceed the number building ecophages by many orders of magnitude. A mix of defenses should be deployed, preferably by multiple agencies to minimize the risk of infiltration.
Some of these defenses should be announced publicly in order to allow a hacker community to try their strength against them, as is common with modern computer security, while other defenses should be kept secret to avoid their circumvention. In this case, the total barrier of a multilayer defensive system like NanoShield should be sufficient to prevent the effective malevolent use of self-replicating nanosystems, and should provide an excellent first line of defense against the threat of even more potent nanoweaponry.
However, it is not necessary to implement the entire NanoShield plan to be reasonably protected against ecophagic attacks. Even a partial implementation would greatly increase the odds that an ecophagic or nonreplicating nanoweapon attack would leave some survivors and would easily be able to handle the bioweapon and pandemic problems that the BioShield proposal [1] was developed to handle.
The reason the NanoShield could handle bioweapons and pandemic problems is that the NanoShield would be designed to handle a large range of designs, from carbon-based to silicon-based to boron-based, to ecophages with virtually no onboard intelligence, as well as those with onboard sophisticated computers, all-mechanical inner workings or designs that include electronic components, etc. In contrast, bioweapons and pandemics would have a much smaller range of designs and therefore be easier for the NanoShield to defeat.
Notes and References
- Lifeboat Foundation Scientific Advisory Board, Lifeboat Foundation BioShield, July 2006.
- Robert A. Freitas Jr., Ralph C. Merkle, Kinematic Self-Replicating Machines, Landes Bioscience, Georgetown, TX, 2004.
- Robert A. Freitas Jr., Some Limits to Global Ecophagy by Biovorous Nanoreplicators, with Public Policy Recommendations, Zyvex preprint, April 2000.
- Hans Moravec and Jesse Easudes, Fractal branching ultra-dexterous robots, NASA: Advanced Concepts Research Projects, January 1999.
- United States Computer Emergency Readiness Team (US-CERT).
- The Tsunami Warning System.
- The Spaceguard Central Node. See also: Spacewatch Project.
- EOSDIS Earth Observing System Data Information System.
- B. Lobitz, L. Beck, A. Huq, B. Wood, G. Fuchs, A.S.G. Faruque, R. Colwell, Climate and infectious disease: Use of remote sensing for detection of Vibrio cholerae by indirect measurement, Proc. Natl. Acad. Sci. (USA) 97(2000):1438–1443.
- Philippe Van Nedervelde and the Lifeboat Foundation Scientific Advisory Board, Lifeboat Foundation SecurityPreserver, 2006.
- Robert A. Freitas Jr., Economic Impact of the Personal Nanofactory, Nanotechnology Perceptions: A Review of Ultraprecision Engineering and Nanotechnology 2(May 2006):111–126.
- Eric D. Isaacs, X-ray nanovision, Nature Vol. 442 (July 6, 2006):35.
- Ray Kurzweil, The Singularity is Near, Penguin Books, New York, NY 2005.