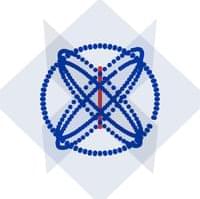
The tin-vacancy center in diamond has properties that could be useful for quantum networks.
In a new study, researchers show how this defect’s electron spin can be controlled — and coherence prolonged — using a superconducting microwave waveguide.
Even the most pristine diamonds can host defects arising from missing atoms (vacancies) or naturally occurring impurities. These defects possess atomlike properties such as charge and spin, which can be accessed optically or magnetically. Over the past few decades, researchers have studied various defects to understand and harness these properties. One in particular—the tin-vacancy center, in which a tin atom resides on an interstitial site with two neighboring vacancies—exhibits exceptionally useful optical and spin properties, making it highly relevant in the field of quantum communication. Here, we explore how the spin properties behave under different magnetic field directions.
We demonstrate that manipulating electron spins is more straightforward in strained diamonds, as the electron spin is more responsive to an alternating magnetic field. We use superconductors known for generating no heat when a current flows through them, ensuring that we do not negatively affect the spin properties.
Through our simple fabrication steps, we illustrate how the properties of the tin-vacancy center can be fully utilized to advance the field of quantum computing and communication.