Kim, Y., Kim, H., Oh, K. et al. Sci Rep 14, 8,356 (2024). https://doi.org/10.1038/s41598-024-58962-3
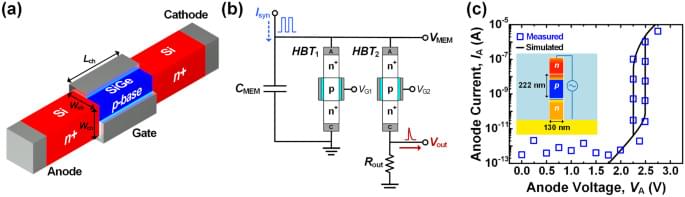
Kim, Y., Kim, H., Oh, K. et al. Sci Rep 14, 8,356 (2024). https://doi.org/10.1038/s41598-024-58962-3
Scientists at Penn State have harnessed a unique property called incipient ferroelectricity to create a new type of computer memory that could revolutionize how electronic devices work, such as using much less energy and operating in extreme environments like outer space.
They published their work, which focuses on multifunctional two-dimensional field-effect transistors (FETs), in Nature Communications. FETs are advanced electronic devices that use ultra-thin layers of materials to control electrical signals, offering multiple functions like switching, sensing or memory in a compact form.
They are ferroelectric-like, meaning the direction of their electric conduction can be reversed when an external electric field is applied to the system. FETs are essential in computing, since the ferroelectric-like property allows them to shift signals.
Silicon is the best-known semiconductor material. However, controlled nanostructuring drastically alters the material’s properties. Using a specially developed etching apparatus, a team at HZB has now produced mesoporous silicon layers with countless tiny pores and investigated their electrical and thermal conductivity.
For the first time, the researchers elucidated the electronic transport mechanism in this mesoporous silicon. The material has great potential for applications and could also be used to thermally insulate qubits for quantum computers. The work is published in Small Structures.
Mesoporous silicon is crystalline silicon with disordered nanometer-sized pores. The material has a huge internal surface area and is also biocompatible. This opens up a wide range of potential applications, from biosensors to battery anodes and capacitors. In addition, the material’s exceptionally low thermal conductivity suggests applications as thermal insulator.
Researchers have developed a battery that can convert nuclear energy into electricity via light emission, a new study suggests.
Nuclear power plants, which generate about 20% of all electricity produced in the United States, produce almost no greenhouse gas emissions. However, these systems do create radioactive waste, which can be dangerous to human health and the environment. Safely disposing of this waste can be challenging.
Using a combination of scintillator crystals, high-density materials that emit light when they absorb radiation, and solar cells, the team, led by researchers from The Ohio State University, demonstrated that ambient gamma radiation could be harvested to produce a strong enough electric output to power microelectronics, like microchips.
Using an approach called DNA origami, scientists at Caltech have developed a technique that could lead to cheaper, reusable biomarker sensors for quickly detecting proteins in bodily fluids, eliminating the need to send samples out to lab centers for testing.
“Our work provides a proof-of-concept showing a path to a single-step method that could be used to identify and measure nucleic acids and proteins,” says Paul Rothemund (BS ‘94), a visiting associate at Caltech in computing and mathematical sciences, and computation and neural systems.
A paper describing the work recently appeared in the journal Proceedings of the National Academy of Sciences. The lead authors of the paper are former Caltech postdoctoral scholar Byoung-jin Jeon and current graduate student Matteo M. Guareschi, who completed the work in Rothemund’s lab.
Researchers developed a theoretical model that predicts a substantial increase in the brightness of organic light-emitting diodes (OLEDs) by leveraging novel quantum states called polaritons. Integrating polaritons into OLEDs effectively requires the discovery of new materials, making practical implementation an exciting challenge.
OLED technology has become a common light source in a variety of high-end display devices, such as smartphones, laptops, TVs or smart watches.
While OLEDs are rapidly reshaping lighting applications with their flexibility and eco-friendliness, they can be quite slow at converting electric current into light, with only a 25% probability in emitting photons efficiently and rapidly. The latter is an important condition for boosting the brightness of OLEDs, which tend to be dimmer than other light technologies.
Quantum light sources are fickle. They can flicker like stars in the night sky and can fade out like a dying flashlight. However, newly published research from the University of Oklahoma proves that adding a covering to one of these light sources, called a colloidal quantum dot, can cause them to shine without faltering, opening the door to new, affordable quantum possibilities. The findings are available in Nature Communications.
Quantum dots, or QDs, are so small that if you scaled up a single quantum dot to the size of a baseball, a baseball would be the size of the moon. QDs are used in a variety of products, from computer monitors and LEDs to solar cells and biomedical engineering devices. They are also used in quantum computing and communication.
A research study led by OU Assistant Professor Yitong Dong demonstrates that adding a crystalized molecular layer to QDs made of perovskite neutralizes surface defects and stabilizes the surface lattices. Doing so prevents them from darkening or blinking.
You may recognize graphite as the “lead” in a pencil, but besides helping you take notes or fill in countless bubbles on exam answer sheets, it is helping scientists grapple with the secrets of superconductivity.
Superconductivity happens when an electric current is transmitted through wires without the loss of any energy in the form of heat or resistance. Superconducting materials have the potential to revolutionize many aspects of our daily lives, from improving the electrical grid to making more powerful computers.
However, superconductivity generally requires very low temperatures, so low they may become impractical, and the exact mechanisms of superconductivity are not well understood for many superconducting materials.
In the late 1960s, physicists like Charles Misner proposed that the regions surrounding singularities—points of infinite density at the centers of black holes—might exhibit chaotic behavior, with space and time undergoing erratic contractions and expansions. This concept, termed the “Mixmaster universe,” suggested that an astronaut venturing into such a black hole would experience a tumultuous mixing of their body parts, akin to the action of a kitchen mixer.
S general theory of relativity, which describes the gravitational dynamics of black holes, employs complex mathematical formulations that intertwine multiple equations. Historically, researchers like Misner introduced simplifying assumptions to make these equations more tractable. However, even with these assumptions, the computational tools of the time were insufficient to fully explore the chaotic nature of these regions, leading to a decline in related research. + Recently, advancements in mathematical techniques and computational power have reignited interest in studying the chaotic environments near singularities. Physicists aim to validate the earlier approximations made by Misner and others, ensuring they accurately reflect the predictions of Einsteinian gravity. Moreover, by delving deeper into the extreme conditions near singularities, researchers hope to bridge the gap between general relativity and quantum mechanics, potentially leading to a unified theory of quantum gravity.
Understanding the intricate and chaotic space-time near black hole singularities not only challenges our current physical theories but also promises to shed light on the fundamental nature of space and time themselves.
Physicists hope that understanding the churning region near singularities might help them reconcile gravity and quantum mechanics.