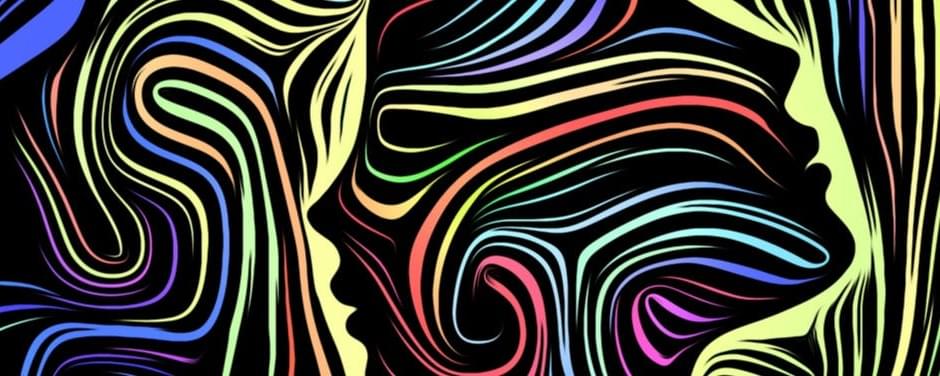
The combination problem may, in fact, be a reason to favor a version of panpsychism in which consciousness is fundamental in the form of a continuous, pervasive field, analogous to spacetime. Just as spacetime and gravity have an interactive relationship, consciousness can be thought of as a fundamental “field” that interacts with, and is integral to, matter. We typically don’t think of spacetime as bits and pieces that build on each other (it’s simply everywhere), and I don’t think we should be tempted to think of consciousness, if it is indeed a pervasive field, as divisible into building blocks either. Rather, it makes more sense to talk about a field that contains a range of content —the content depending on the other forces or fields it’s interacting with. In the same way that gravity is a two-way street—matter warps spacetime and the shape of spacetime determines how matter moves—a consciousness field would imbue matter with another property, giving rise to the range of content experience d. Under this view, content is divisible, but consciousness isn’t. Therefore, consciousness is also not interacting with itself, as it would be in the act of “combining.” Considering consciousness to be fundamental allows for matter to have a specific internal character everywhere, in all of its various forms.
If consciousness is fundamental, then the questions that prompt the combination problem are potentially the same as all the other questions we might ask about spacetime in which we don’t anticipate this problem. All matter would entail consciousness, and complex systems, such as human brains, would give rise to certain types of content in those locations in spacetime. Even if each individual atom has its own experience, consciousness itself is not necessarily isolated. The matter might be isolated, and therefore the content associated with the consciousness at that location is isolated. But consciousness itself would not be said to be isolated. Again, we can think of consciousness as analogous to spacetime: How it’s affected by matter depends on the matter in question (its mass, in the case of spacetime). Similarly, a consciousness field might be “shaped” by matter in terms of experiential quality or content. And this line of thinking yields interesting questions.